
Research
High-Frequency Sensor Networks:
Monitoring Our Dynamic
Aquatic Environments
Environmental sensors are becoming more accurate and reliable; the cost of transmitting and storing data is plummeting; and the diversity and power of the tools to disentangle complex environmental signals is rapidly improving. But data science alone will not revolutionize environmental monitoring and research. Networks of sensors must be carefully designed and deployed at the scales necessary to capture the relevant processes. Which processes do we expect to be relevant? How do we ensure that our data represent true environmental signals? Theory, observation, and experience guide well-formed hypotheses and monitoring approaches, intelligent data curation, and insightful analyses.
​
I design and manage sensor networks in aquatic environments to develop data products that allow myself and other researchers to:
-
Establish baseline water quality conditions.
-
Explore trends in our changing environment.
-
Assess the dominant water quality processes.
-
Guide experimental design.
​
Example research projects associated with these data collection efforts are described below.
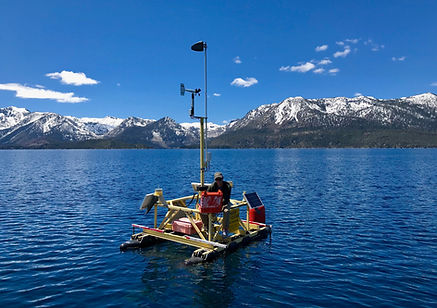
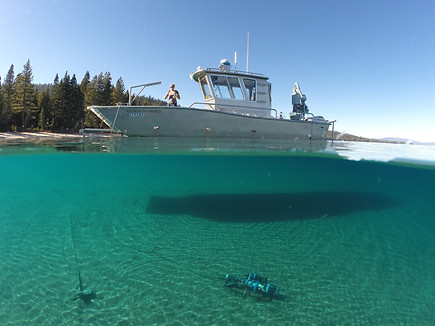
Photo: Brant Allen (UC Davis)
Photo: Brant Allen (UC Davis)
Lake Upwelling:
The Mechanics and Impacts of Upwelling in Lake Tahoe
Sustained winds can draw cold and often nutrient-rich water to the lake surface from depth. At Lake Tahoe, the prevailing southwesterly winds drive a predictable upwelling pattern along the west shore in late spring. Our observations show upwelling from deeper than 70 m, making Tahoe's stratified-season upwelling pattern among the largest magnitude observed in any lake. However, because Tahoe's nutrients are stored exceptionally deep, these upwelling events are rarely large enough to increase near-surface nutrient concentrations. Interestingly, it is when the winds subside, setting off a suite of internal waves, that the lake sees the strongest currents, which can exceed 25 cm/s.
​

The setup and relaxation of spring upwelling in a deep, rotationally influenced lake.
Seasonal upwelling patterns drive water quality heterogeneity in the nearshore of a deep lake. (In prep)
Associated publications:
3D Flow Structures During Upwelling Events in Lakes of Moderate Size. Water Resources Research (2022)
Estuarine Metabolism:
Oxygen Dynamics in the Tidal Sloughs of San Francisco Bay
Dissolved oxygen concentrations in estuaries represent complex balances of physical and biogeochemical processes. In the tidal sloughs of Lower South San Francisco Bay, these balances can shift rapidly and between seasons, responding to tides, water temperature, and biological cycles, among other factors. In summer, when the highest tides align with nighttime hours, strong oxygen demand associated with over-marsh flooding cannot be balanced by primary production, leading to open-bay oxygen minima on the subsequent low tides. In and around the highly productive restored salt ponds of the peripheral Lower South Bay, in-pond biomass production drives peak oxygen demand in the surrounding turbid sloughs.
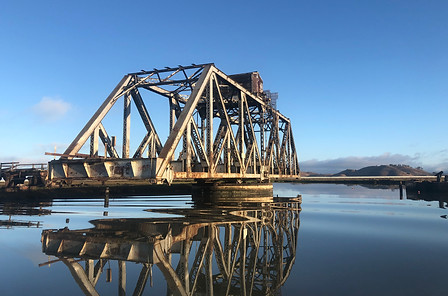
Connections to tidal marsh and restored salt ponds drive seasonal and spatial variability in ecosystem metabolic rates in Lower South San Francisco Bay. Estuaries and Coasts (2022)
Associated publications:
Darkened skies from wildfire smoke, extreme heat trigger hypoxia in the San Francisco Estuary. (In prep)
Nearshore Waves and Sediment:
Prevailing wind patterns drive water clarity at the perimeter of Lake Tahoe
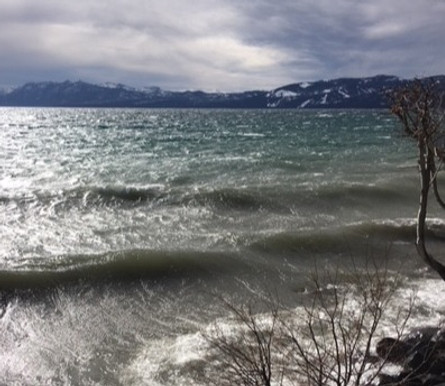

Predicting wave-induced sediment resuspension at the perimeter of lakes using a steady-state spectral wave model.
Wind waves are among the most vigorous hydrodynamic features at the perimeter of lakes. Waves resuspend sediments, affecting light penetration through the water column and, thus, biological processes. Wave-driven flows can increase pore-water flow, catalyzing nutrient delivery to algae, but can also tear attached algae from shallow benthic surfaces. We generated a set of steady-state wind-wave model output for a range of wind conditions, then used a simple table-referencing algorithm to efficiently emulate model simulations. Our results, eight years of hourly wave conditions throughout Lake Tahoe's nearshore, add detail to intuitive patterns associated with the prevailing southweterly winds, but also highlight significant interannual variability in wave forcing.
​
Associated publications:
Surface Seiches:
How is a lake like a guitar string?

A string strung across a guitar will oscillate at the same frequency independent of the magnitude of its oscillations; an E string will sound an E regardless of how hard you pluck it. Lakes, like guitar strings, are natural harmonic oscillators. As long as the basin shape and water depth remain approximately constant, lakes will "slosh" back and forth at the same frequency in response to perturbations of a variety of magnitudes - winds, atmospheric pressure fronts, or even earthquakes. The structure of this "sloshing" can be surprisingly complex; the multi-dimensionality of a lake (compared to a guitar string) can support superimposed oscillations, known as surface seiches, with different shapes and frequencies. In Lake Tahoe, the periods of these oscillations range between 10 and 30 minutes. Although the magnitude of Tahoe's surface seiches is generally small (less than 5 cm), they can play outsized roles in driving exchange flow with peripheral water bodies, such as Emerald Bay.
​
Observations and modeling of the surface seiches of Lake Tahoe, USA.
Associated publications:
Snowmelt Inflows:
A warming climate may alter the way streams mix into Lake Tahoe
A warming climate is shifting the rain-snow elevation boundary upwards in the Sierra Nevada, CA/NV. At about 6220 ft. above sea-level, Lake Tahoe is situated close to this boundary. Warmer winters increase the proportion of precipitation that falls as rain compared to as snow, leading to a smaller snowpack and an earlier snowmelt. Due to Lake Tahoe's enormous volume, lake temperatures are largely unaffected by snowmelt timing and quantity. Warmer winters shift peak snowmelt earlier, and do so more than they shift the timing of spring lake warming. Thus, in warmer winters, when peak snowmelt happens earlier, cold inflows enter a cold lake, tend to be neutrally buoyant and, as a result, may largely be mixed into the surface waters nearshore. In colder winters, more precipitation falls as snow and peak snowmelt happens later, when the lake has begun to warm. As a result, peak inflows tend to be colder than the warmer receiving lake waters, are comparatively dense, and are more likely to plunge to depth.
Snowmelt timing as a determinant of lake inflow mixing.


Associated publications: